Ⅰ. INTRODUCTION
Satellite Synthetic-Aperture Radar(SAR) systems require high-performance antennas for optimal operation. Additional weight and profile restrictions to antennas exist in addition to those comparable terrestrial or airborne radar/SAR systems. Microstrip patch arrays have exceptionally low profiles and weights relative to their waveguide or reflector antenna counterparts, making them attractive platforms for spaceborne SAR antennas; they have been recently used extensively in the industry[1]∼[3]. Both single- and dual-polarization antennas have been used in SAR systems. The dual polarization system has an advantage over the standard single polarization antenna, in that it can obtain polarimetric data, thus increasing the pool of information that can be extracted from the target[4].
In this work, a dual linearly polarized microstrip patch array is presented. The antenna has 2×4 radiation elements and operates in X-band, with the center frequency denoted as f0. The antenna is designed to have an impedance bandwidth of more than 12.2% and maximize the gain, with predetermined subarray size of 88.0×44.0 mm2. The antenna is intended to be used as a subarray module in a large-scale array for SAR application. The antenna’s total size is 23×23 wavelengths (at the center frequency f0) in size and has estimated gain of more than 35 dBi. A large array based on a small subarray reduces the overall complexity of the antenna, facilitates larger antenna gain, and allows for beam scanning.
Two independent stripline corporate feeding networks located on the same layer are used to distribute power to the dual-polarization radiation elements. The feeding network simultaneously has inherent wideband performance, excellent polarization isolation and low spurious radiation. It also features only 0.6 dB of simulated insertion loss. However, via stitching, it is necessary to suppress the excitation and propagation of parallel plate modes, due to discontinuities in the feeding network. Using an aperture-fed stacked-patch[5],[6] as the radiation element and a relatively thick honeycomb substrate, a wide impedance bandwidth, in excess of 19 % at Return Loss (RL) level < −10 dB, is obtained. The peak realized gain of the subarray is measured as 15.7 dBi, and the XpD is more than 40 dB.
Ⅱ. ANTENNA DESIGN
Multiple different techniques exist for impedance bandwidth improvement in microstrip patch antennas. Some of them include utilizing thick low-permittivity substrates, multiple coupled resonant elements, co-planar parasitic elements, reactive loading, or variously shaped slots cut in the microstrip patch element. In this study, a coupled stacked patch configuration separated by a thick low-permittivity honeycomb substrate is used. Using this combination, a very wide impedance bandwidth can be obtained. The large separation between the patches ensures low-coupling coefficient between the two patch elements, resulting in closely spaced resonant peaks in the input impedance of the structure.
A small H-shaped modified aperture[7] located in the groundplane separating the feeding network and lower patch element is used to couple power to the radiation elements. The substrate used between the lower patch and coupling aperture is relatively thin and has high permittivity; thus, a high coupling coefficient can be achieved for a relatively small aperture size. Small apertures have extremely reactive impedance and do not contribute to radiation. The aperture functions as a step-down transformer, transforming the high input impedance of the patch antenna down to an impedance comparable to that of the transmission lines used in the feeding network.
The designed 2×4 microstrip array is constructed of five dielectric and five conductive layers. The stripline feeding network of two Taconic RF-35[8] dielectric laminate substrates surrounded by two ground planes. The substrate has a nominal dielectric constant of 3.5 and a loss tangent of 0.0018. Similarly, the two radiation patch elements are made on the RF-35 substrate; however, they are separated by an Ultracor UQF-105-1/4[9] honeycomb dielectric layer. This honeycomb material exhibits a dielectric constant near unity, and has an extremely low dielectric loss factor of less than 0.00083. The honeycomb substrate enables wideband impedance performance and the minimization of dielectric loss in the antenna. Additionally, the use of a honeycomb substrate, instead of standard solid dielectric materials, allows for a significantly lower antenna weight[10],[11]. The antenna’s PCB stack-up adopted in this study is shown in Fig. 1.
Blind via holes are used to stitch layers #3 and #5, which are the ground planes forming the stripline feeding network. This is done to provide excellent shielding between the two feeding networks, and suppress the excitation of the parallel plate mode by discontinues in the stripline[12],[13].
The antenna is designed, and its performance is verified using a commercial electro-magnetic simulator ANSYS HFSS. The simulation model used is shown in Fig. 2. The feeding networks are color-coded as green representing the feeding network facilitating vertically polarized radiation, and red for that of horizontally polarized radiation. The detailed normalized dimensions of the radiation element unit cell are shown in Fig. 3.
The radiation elements are fed via two orthogonally located apertures placed directly under the radiation elements. Each aperture is fed by the H- or V-pol feeding network. Any coupling between the closely spaced apertures results in an out-of-phase summation within the feeding network of the opposing polarization, resulting in very high isolation between the two polarizations. Fig. 4 shows the current distribution J, on the upper patch of the assembly when only one of the feeding networks is excited.
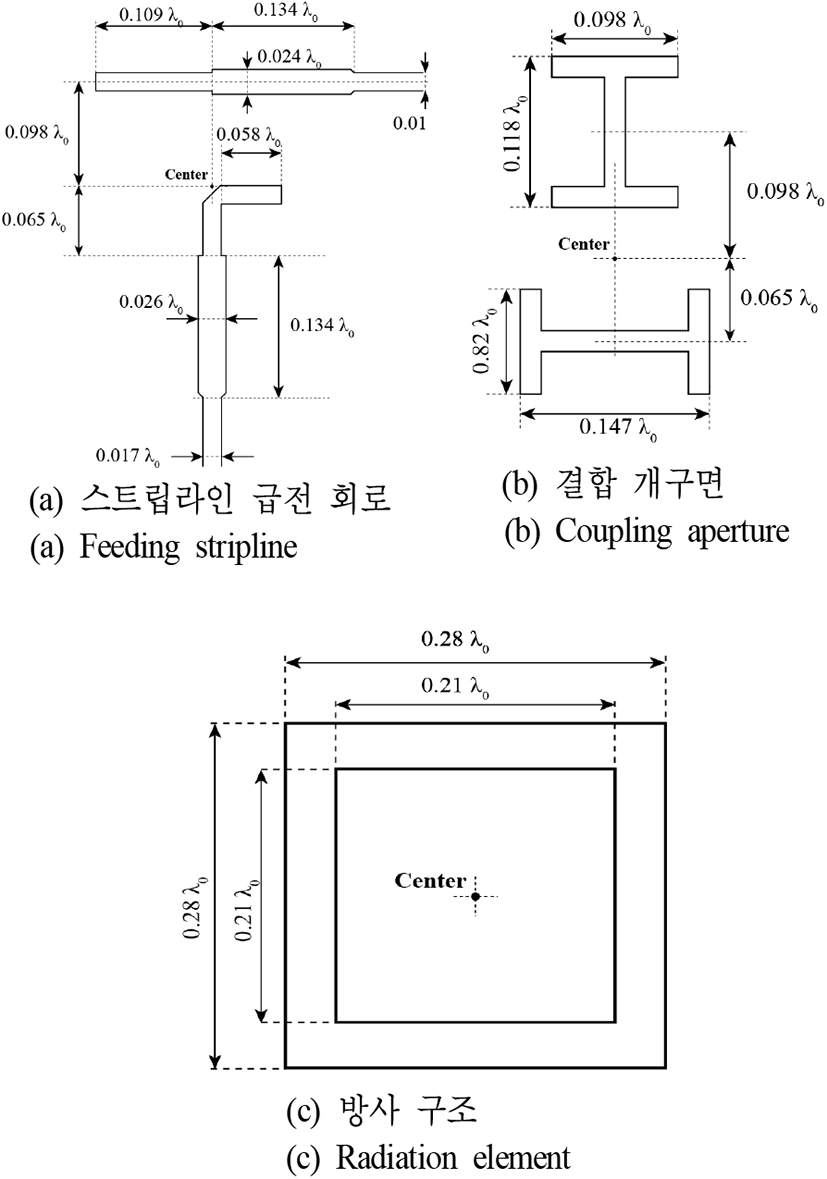
Ⅲ. SIMULATION AND MEASUREMENT RESULTS
The antenna is fabricated via a standard PCB manufacturing process. The total size of the PCB assembly is 88.0× 44.0×5.1 mm3. Two surface mount SMA-F connectors are used to feed the antenna. The antenna’s RL and isolation are measured using a two-port Anritsu 37247D 20 GHz Vector Network Analyzer (VNA). The radiation pattern and gain of the antenna are measured using a tapered anechoic chamber, MTG antenna measurement system and Agilent Technologies E8364B 50 GHz VNA. Images of the fabricated antenna are shown in Fig. 5.
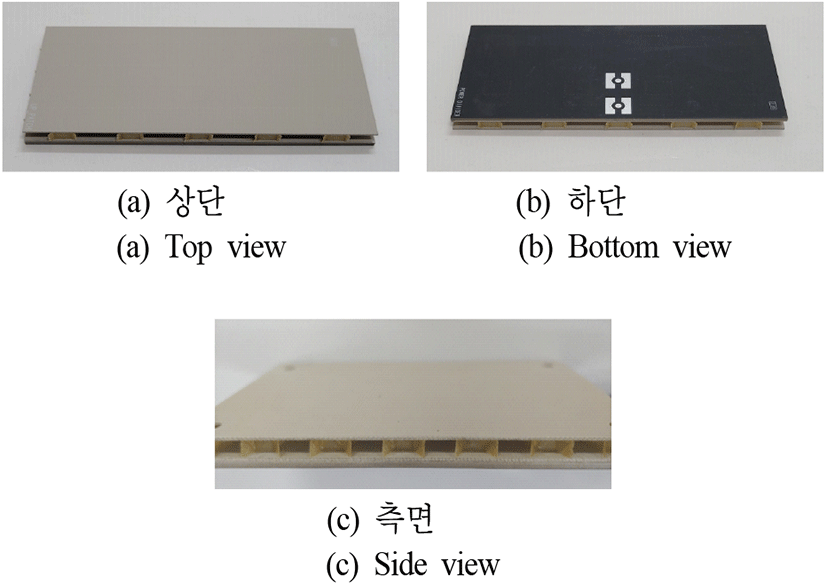
A comparison between the measured and simulated RL of the antenna is presented in Fig. 6. The antenna features measured −10 dB RL bandwidth for H- and V-pol of 19.4 % and 21.1 % respectively. Slight frequency shift in the measured impedance bandwidth relative to the simulated RL can be observed. The frequency shift can be caused by the change in the electrical length of components in the feeding network, because of variations in the dielectric constant of the substrate[8]. Moreover, the center frequency of patch antennas is very sensitive to substrate height variations. Bonding film is used to attach the feeding network and stacked patch assemblies, causing significant enough change in height, enough to offset the center frequency. Additionally, the coupling between the two antenna ports is measured as less than −55 dB within the band of interest.
Fig. 7 and Fig. 8 show a comparison of the simulated and measured radiation patterns. Good agreement between the datasets is observed. Respective antenna beam widths of 41°/19° in the elevation and azimuth planes are measured for V-pol radiation, and 37°/18° for H-pol radiation. The obtained Side Lobe Level (SLL) is −12.3 dB for V-pol and −12.6 dB for H-pol. The non-ideal amplitude distribution and mutual coupling between elements causes the SLL to be higher than the ideal value of −13.3 dB.
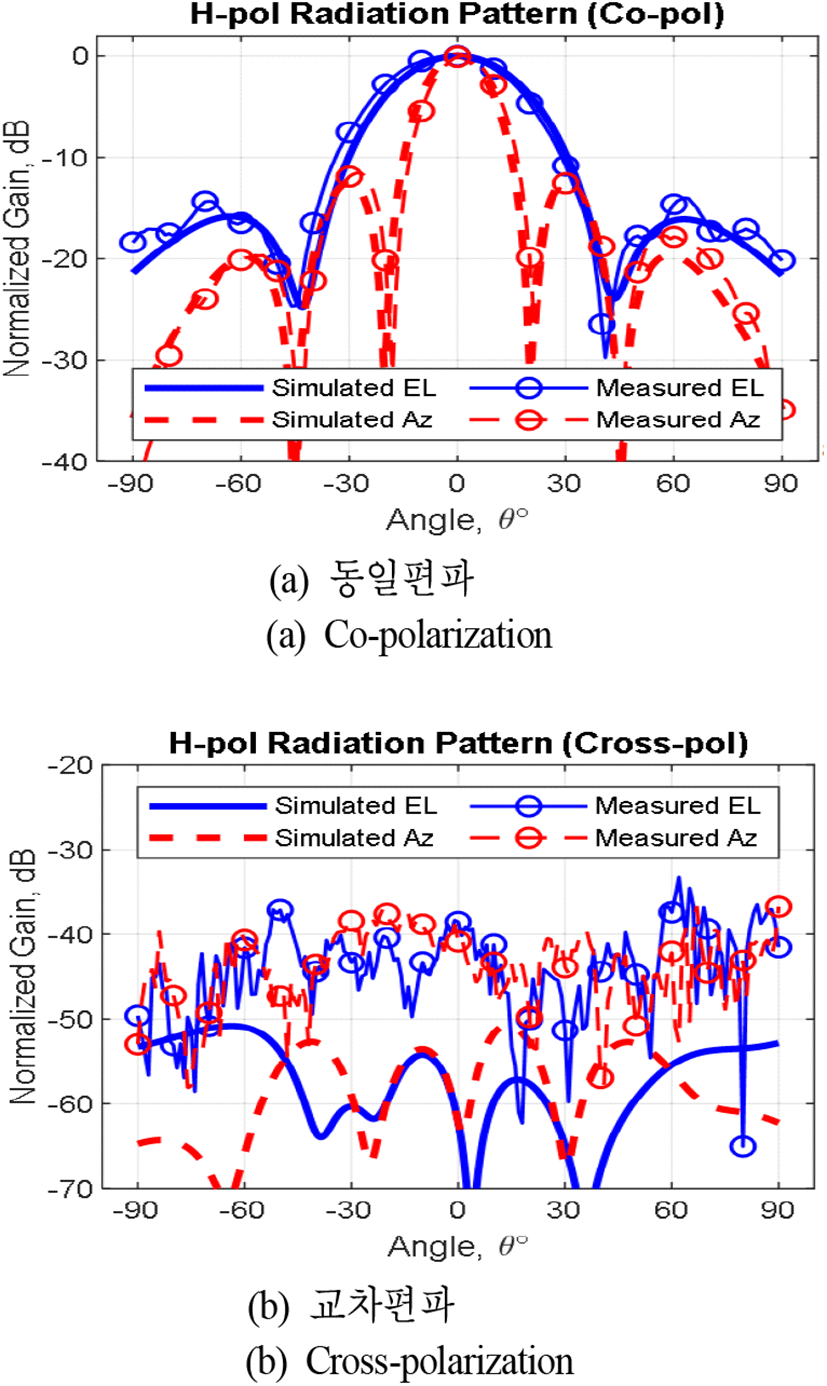
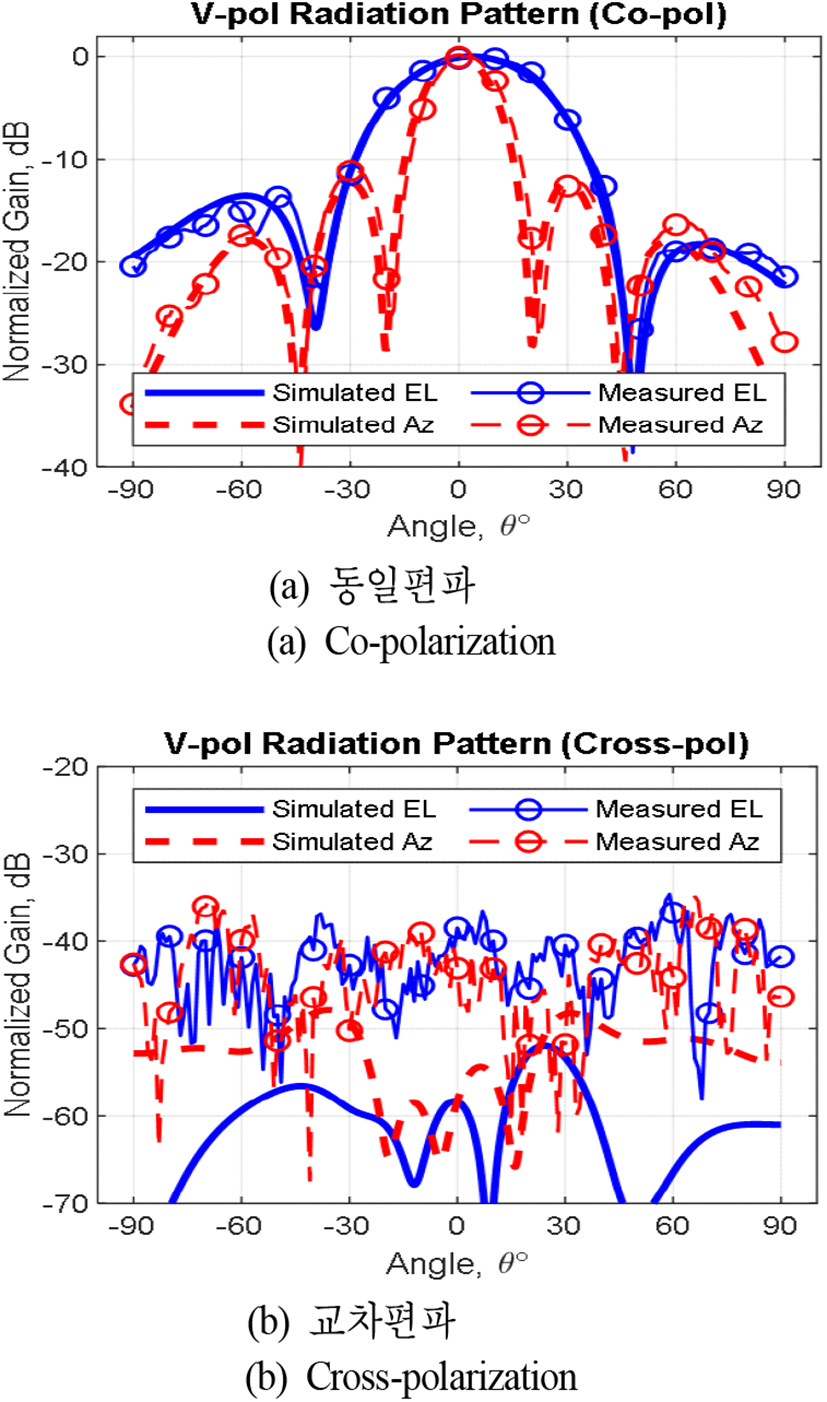
The peak measured gain for H-pol is 15.7 dBi, and that for V-pol 14.8 dBi, as shown in Fig. 9. The measured XpD level is 40 dB for both polarizations, where the simulated value is less than 55 dB. The XpD measurement is limited by the noise performance of the measurement system. Owing to the small dynamic range of the system the measured value of XpD corresponds to the noise floor of the VNA.
The performance of the proposed antenna is compared with two other works for SAR applications that utilize microstrip patch as the radiation element. A parameter-wise comparison between the antennas is presented in Table 1. The proposed design exhibits a significantly larger −10 dB impedance bandwidth compared with the other two works. Because [14] does not utilize any parasitic elements in the antenna design, the bandwidth is extremely limited. The largest reported impedance bandwidth in [13] is 11.8 % at RL level of −14 dB, and in [14] of 3 % at −18 dB RL level. It can be seen from [13] and [14] that the −10 dB bandwidth is in the range of only 13 % and 7.1% respectively, compared to the proposed 21.1%. The proposed antenna has significantly higher isolation and XpD than the other two works. This is mainly due to the use of stripline in the feeding network. The via stitching around the stripline limits the coupling between the two polarizations and improves both the isolation and Cross-Polarization Discrimination (XpD) performance of the antenna. The SLL level between the proposed antenna and [13] differs significantly. In this work, the array is uniformly excited, whereas in [13], aperture taper is applied reducing the SLL level and gain.
Ⅳ. CONCLUSION
In this work, a 2×4 element dual linearly polarized stacked patch antenna array is presented. A stripline feeding network is used to provide excellent cross-polarization performance and isolation between the two polarizations. An antenna prototype is manufactured and its impedance and radiation performance are quantified. A wide impedance bandwidth of 21.1 % at an RL level of −10 dB. The antenna exhibits 15.7 dBi gain, XpD in excess of 40 dB and SLL of less than −12.3 dB.